Research
What I do
I study the formation and evolution of galaxies using primarily observational techniques. I try to determine how galaxies assembled over cosmic time and how their evolution is affected by the places in which they reside as they evolve, what we call their "environments". I address these research questions by using a variety of telescopes on the ground and in space to observe galaxies at most wavelengths and everywhere from the very nearby Universe to so far away that light left those objects 10 billion years in the past. Doing so I can directly track galaxy evolution and the dependence of that evolution on individual galaxy properties and their surroundings. I am especially interested in how the gas flowing into and out of galaxies is modulated by their environment. Progress in these fields will give us a better idea of how our own galaxy formed and how galaxies in the local Universe today attained their present state.

Research comes from people
At its core, research is a human endeavor. I am lucky to have enthusiastic, compassionate, creative, responsible, and brilliant colleagues and students. Without them, my research wouldn't be possible.Understanding galaxies in distant clusters with the GOGREEN (Gemini Observations of Galaxies in Rich Early ENvironments) survey
I am on the management board of the GOGREEN (Gemini Observations of Galaxies in Rich Early ENvironments) survey, which is the premier spectroscopic survey of clusters and groups of galaxies at z>1 conducted with the largest telescope publicly available to US astronomers. The goals of this survey were to uncover how environment quenches star formation and transforms galaxies. We also learned how the stellar content of dark matter halos has evolved over time.

1500 spectra of distant galaxies
All GOGREEN spectra, ordered in increasing redshift. This image has a reverse color scheme such that the dark regions indicate brighter emission. The redshift range of the GOGREEN clusters is indicated, as well as the absorption (light) and emission (dark) lines. The ultradeep GOGREEN spectra allow us to measure redshifts of passive galaxies out to z=1.5 (Balogh et al. w/ Rudnick 2021). The publications from the survey can be found here
GOGREEN clusters have an excess of transition galaxies
GOGREEN clusters have an excess of transition galaxies that are quenching their star formation. This excess increases towards lower stellar masses. (McNab et al. with Rudnick 2021)Galaxies traversing the cosmic web in the Virgo Filament Survey (VFS)
I am a lead member of the VFS, which is exploring galaxies in the very distributed network of filaments surrounding the Virgo Cluster. Our goal is to understand how the baryon cycle in galaxies and galactic star formation rates and structure are modified as galaxies pass through the cosmic web. We are carrying out a multiwavelength study of the dust, molecular gas, neutral hydrogen, and ionized gas through a broad set of observations including those with MeerKAT, ALMA, IRAM, NOEMA, and a large Halpha survey of ~650 galaxies.

The filaments around the Virgo Cluster
This figure from Castignani et al. w/Rudnick (2022) shows the location of the filaments in the VFS that are located around the Virgo Cluster and extend up to 12 virial radii away from the cluster.How star formation is being modified in galaxies around Virgo
My graduate student Kim Conger has used 12 micron emission from the WISE satellite to measure the extent of star-forming disks in galaxies residing a large range of densities around the Virgo cluster. She finds that the extent of star-forming disks in cluster and filament galaxies are smaller compared to those in field galaxies. She also compares her results to state-of-the-art semi-analytic models (SAMs) of galaxy formation. She finds that the models predict the size of field galaxies well, but underpredict the extent of star formation in dense environments. These same models, however, predict the correct fraction of galaxies with no star formation, implying that their implementation of environmental effects is incomplete.
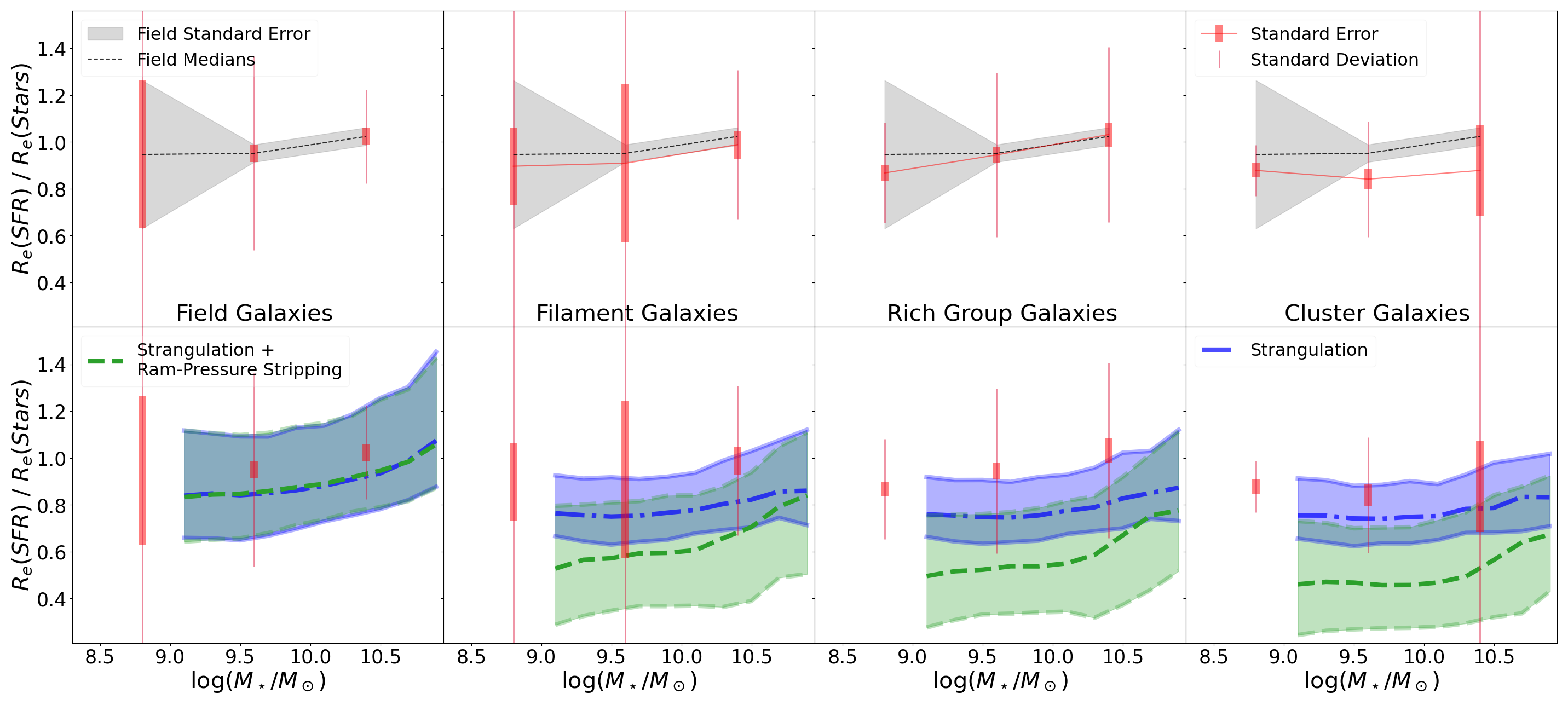
The molecular gas contents and star formation rate in distant cluster galaxies
If we want to understand how the oldest present-day cluster galaxies stopped forming stars, we need to observe the progenitors of these galaxies in distant clusters. A specifically powerful approach is to study the molecular gas contents of these galaxies, as this gas is the fuel for star formation. I work with colleagues, including Allison Noble at ASU, to understand how the molecular gas is distributed in clusters 9 Billion Light years away. Using extremely deep and high resolution data from the Atacama Large Millimeter Array (ALMA) we have been able to observe that these distant cluster galaxies are overabundant in their molecular gas compared to similarly star-forming galaxies in the field. We also have been able to determine that the spatial distribution of gas in these galaxies shows striking asymmetries, akin to what is found in much more mature local clusters. This indicates that even these distant and immature clusters may be able to alter the gas contents of the galaxies that lie within them.
I am leading efforts to obtain a large census of the molecular gas density in the GOGREEN and GCLASS clusters described above.
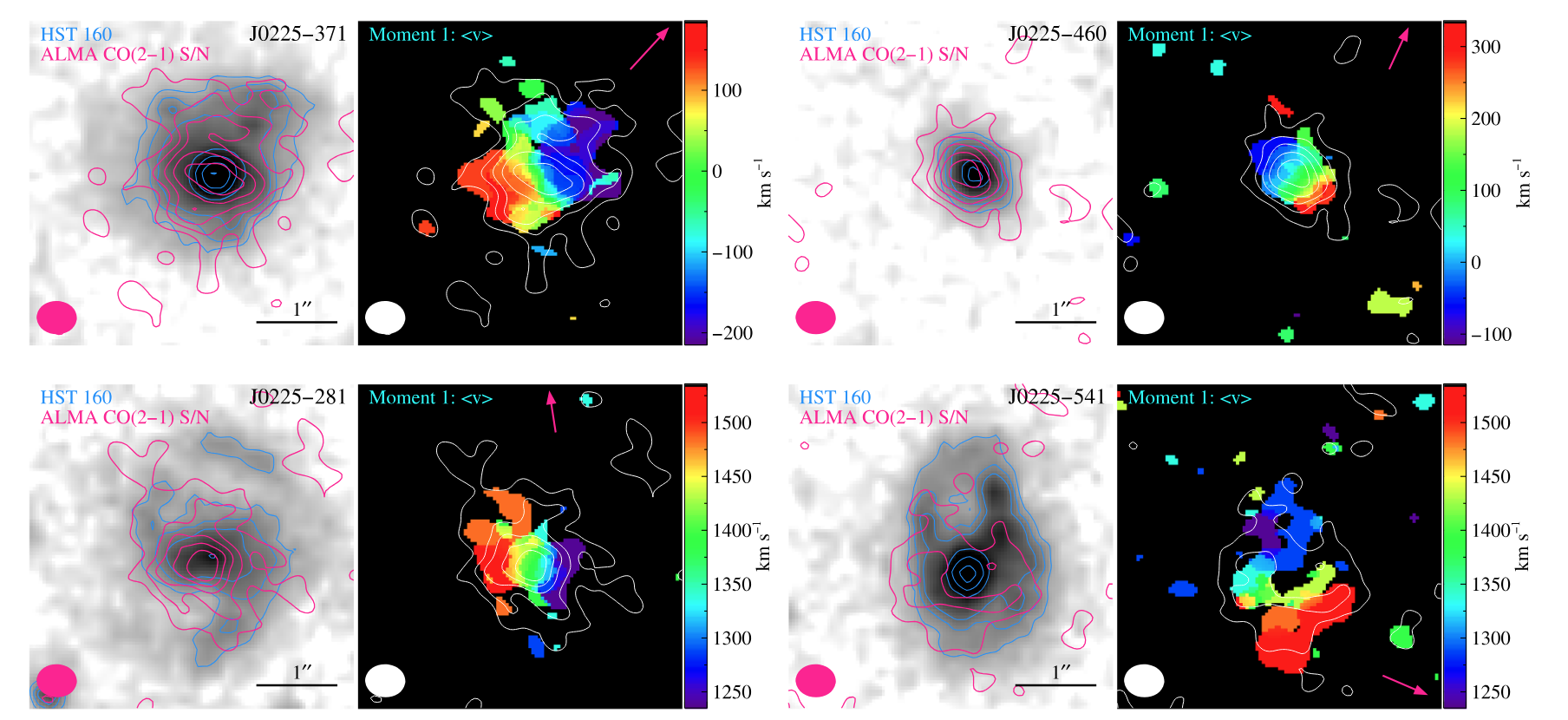
Using deconvolution to study the spatially resolve properties of distant galaxies
We are at the limit of what we can learn from spatially integrated images of galaxies. While observations with JWST are revealing fascinating details about distant galaxies, these surveys are limited both in their wavelength coverage and in their area. Recent advancements in mature deconvolution techniques allow us to sharpen ground-based images using our knowledge of how the images are blurred by the earth's atmosphere. These techniques operate with no prior knowledge of how the true image looks and the newest developments have been able to improve ground-based image quality by a factor of ~3.
With these techniques we will be able to understand where galaxies cease their star formation first in dense environments, which will give us new insights into the physical mechanisms that quench star formation.
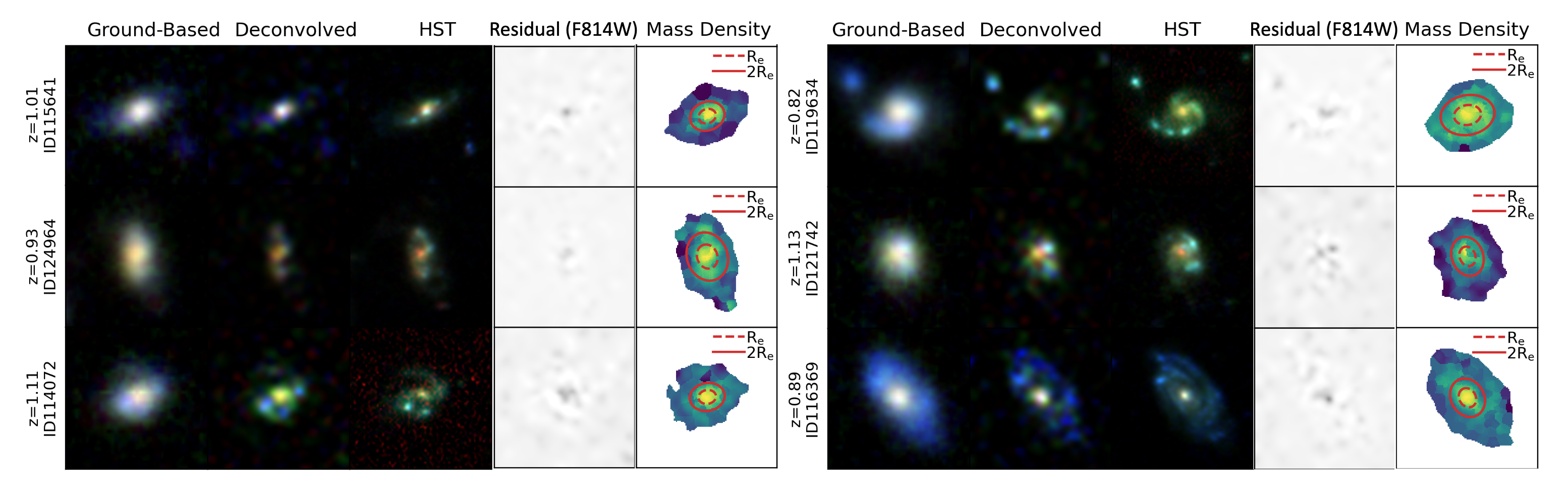
Demonstration of Firedec deconvolution applied to six representative galaxies at z ∼ 1 from the COSMOS/UltraVISTA field (Sok et al. 2021). Shown for each galaxy (left to right) is: ground-based VzH color image (∼0. 8 seeing); the corresponding deconvolved V zH images (FWHM∼0. 3); a color HST image using similar bands (at slightly better FWHM∼0. 2 arcsec); residual flux between the deconvolved z-image and HST/F814W image; stellar mass map derived from spatially resolved SED fitting to the multi-band images. The HST images are used only for comparison and not as input to the deconvolution. The figure shows that the deconvolved images (second column) recover the structure in the HST images (third column) very well (fourth column), without the HST images having been used as a prior. It also reveals striking color gradients in many of the galaxies which are much more pronounced after deconvolution (second column). The GOGREEN images have similar signal-to-noise as these observations. As part of an ongoing NSF grant, we are deconvolving GOGREEN images using an improved technique to determine where quenching occurs within each galaxy and how the stellar mass in each galaxy is distributed.
Applying deconvolution to large surveys
Schematic showing the gains in area and spatial resolution (at 1μm) achievable by deconvolving ground-based multi-passband imaging from current and future surveys (magenta to blue). The deconvolution technique, we are using has already been successfully applied to the COSMOS/UltraVISTA field by our collaborators, resulting in new images with 0.3arcsecond resolution. We are applying the same technique, which requires no morphological priors and can be applied over large fields of view,to the GOGREEN cluster survey dataset, making publicly available multi-passband images with 0.3 arcsecond resolution over a larger area than well known JWST and HST surveys (yellow). This work will serve as a pathfinder for future large ground-based surveys, e.g. VRO/LSST (right).
Interdisciplinary research with Computer Scientists.
Applying deconvolution efficiently and robustly to large datasets requires new computational techniques. I am working with collaborators at EPFL Prof. Pascale Jablonka and Utsav Akhaury to use machine learning to improve the ability to quickly deconvolve large numbers of galaxies. Utsav is a computer science student and brings valuable skills and insight to the project. Future implementations of advanced image processing techniques will require collaboration with computer scientists and other experts in image processing and machine learning.